At Antheia, we spend a lot of time talking about synthetic biology due to the nature of our work engineering yeast to produce active pharmaceutical ingredients (APIs) and key starting materials (KSMs) for essential medicines. However, synbio’s cousin, the long established field of synthetic chemistry, still plays a vital role. This blog is an introduction and primer for a series about synthetic chemistry, where we’ll discuss the ways that synthetic chemistry complements synthetic biology and biopharma manufacturing.
We invite you to follow along as we explore how synthetic chemistry supports us in unleashing the power of nature to discover new medicines and generate a more efficient, resilient, and equitable pharmaceutical manufacturing model.
Synthetic Chemistry, Defined
Broadly speaking, synthetic chemistry is about transforming matter from one compound into another, and it is especially about creating compounds with new properties. Usually when people think of synthetic chemistry or chemical synthesis, glassware, organic solvents, and metal catalysts come to mind. In contrast, synthetic biology and biotechnology evoke images of cells and enzymes, where living cultures are making a product. But here at Antheia we’re very familiar with the fact that these definitions aren’t absolute and, importantly, that we can combine these two technologies.
We would define traditional synthetic chemistry as the physical or chemical manipulation of substances in a controlled lab environment to produce specific chemical reactions that yield desired formulations. Synthetic chemistry often entails a multi-step process that requires successive rounds of purification and processing to produce a final chemical product – this is often referred to as long chemical synthesis. Synthetic biology may also be used to perform long chemical syntheses, as we do in our work at Antheia, though with some differences from what we would call traditional synthetic chemistry. One differentiating feature between chemical synthesis and biosynthesis is that biosynthesis always utilizes biological components in either living or synthetic cells. Synthetic biology also takes advantage of biological components, but can extend beyond the limitations of biosynthesis in natural systems.
In biotechnology, for example, in vitro biocatalysis is the practice of using biologically produced materials, such as enzymes, that have been extracted or purified first, to perform chemical reactions. Which category would such a process fall within? We would argue that it is most appropriately categorized as synthetic chemistry, in that you are adding enzymes to a chemical reaction like you would any other component. The development of engineered enzymes to perform these reactions would constitute synthetic biology, though these reactions would still not be considered biosynthesis because it is done outside of a living cell. Leveraging enzymes for in vitro biocatalysis can offer important advantages over traditional synthetic chemistry pathways, such as catalyzing reactions more quickly, enabling reactions with selectivities that may be otherwise impossible with non-enzyme catalysts, and performing these reactions in environmentally sustainable conditions. But it still has one major disadvantage: you must obtain the enzymes to run the reaction yourself. For manufacturing at scale, large amounts of purified protein that retains its activity is required. This is an expensive, lengthy, and laborious task fraught with potential errors such as protein misfolding and low yields.
There is a saying that the best chemical reaction is the one that you don’t have to do – and unfortunately, synthetic chemistry is defined entirely by reactions that you do. In contrast, biosynthesis puts the cells to work. Using the genes we insert into the cell as templates, the cell produces all of the necessary enzymes, cofactors, and substrates needed, largely from simple sugars, and then performs all of the reactions necessary to produce our product of interest. At Antheia, we draw a fine line here between how we talk about synthetic chemistry and synthetic biology; while these reactions might be making the same products from the same inputs, even at times using the same enzymes to get it done, in synthetic chemistry, scientists must do the work, and in biosynthesis the cells do the work.
Complementary Tools
That fine line is a great thing to highlight for the purposes of this primer. However, highlighting that separation between the two processes – synthetic biology and synthetic chemistry – is less critical in practice. In our biomanufacturing, they are complementary tools that work together harmoniously, so we have little need to categorize them so starkly in our work. Antheia relies on them both, using each tool in order to maximize the potential of the other.
In synthetic biology, some of the plant natural product pathways are dozens of steps long. That means dozens of usually very chemically similar intermediates, often happening all in the same cell simultaneously, as you would see in a plant in nature. Within a cell, you’ll find a soup of similar-looking molecules – each enzyme is able to find and interact with its preferred substrate, and perform a specific reaction, all under the same conditions.
Similarly, when synthetic chemistry is combined with synthetic biology, we find that enzymes can accomplish a lot of things that traditional small molecule catalysts cannot:
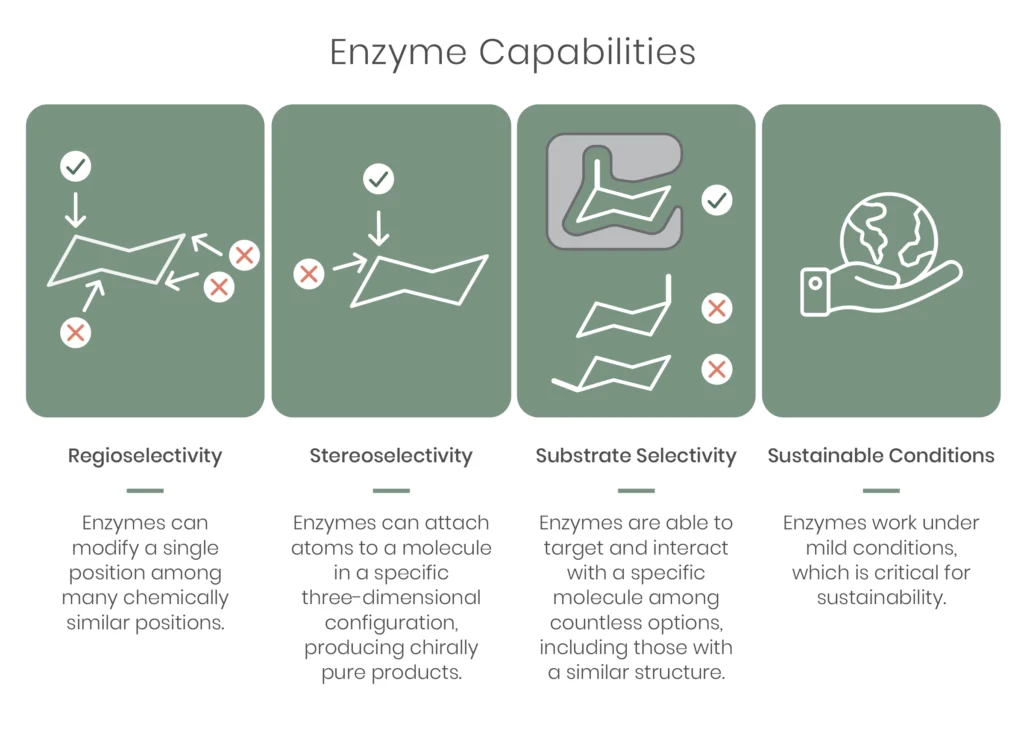
This all equates to a level of efficiency that traditional synthetic chemistry lacks, since without the dance of enzyme-substrate selectivity, teams must cope with individual isolation and purification of lots of intermediates, and also the need to set up each subsequent step, often under very different conditions than the previous step.
This is why many chemists will tell you that the worst part of any synthesis is purification. It is a necessary step because chemical reactions, unlike enzymatic reactions with their substrate selectivity, tend to be relatively non-discriminatory. That means with each round of chemical synthesis, you may end up with increasing numbers of intermediate outputs to separate and purify. Synthetic biology offers huge potential to replace not just one individual step of chemical synthesis with a biosynthetic step – but to replace multiple consecutive steps that would otherwise require laborious purifications and different reaction conditions for each step. With synthetic biology, a cell can do all these steps under the same conditions with no purifications necessary. It is simply one of many appealing examples of where synthetic biology can complement synthetic chemistry, and create transformative efficiency.
Conclusion
Synthetic chemistry and synthetic biology are powerful tools that can complement each other to allow new and easy access to a wider range of molecules than we have ever been able to produce before. While we seek to use synthetic biology to reduce much of the work of synthetic chemistry – by engineering biosynthetic pathways to produce molecules that we previously relied on nature and synthetic chemistry to produce – we don’t view synthetic biology as being in conflict with synthetic chemistry. Instead, synthetic chemistry informs every part of our work at Antheia – we rely on synthetic chemistry to probe and understand the reactions occurring in our yeast cells so that we can engineer the enzymes and strains to work better for us.
In the next installment of Antheia’s Synthetic Chemistry Series, we’ll dive deeper into how synthetic chemistry supports innovation, and begin to evaluate the challenges of synthetic chemistry, and its applications in the pharmaceutical space.